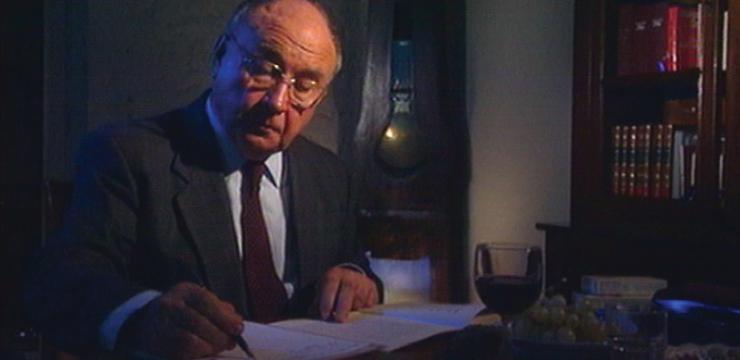
- Excerpt
From 1990 until 2009, the year when he passed away, Prof. Jack Masquelier provided me firsthand knowledge based on decades of scientific investigations that he had performed or meticulously supervised. He also transfered to me stacks of original records. Files, publications and handwritten notes concerning the scientific, intellectual, industrial and commercial history of his OPCs. He took the time to carefully and patiently explain OPCs’ structure, their health effects and the ways in which they function. Among those many documents is an article he published in 1990, the year we first met, in the French “library journal” NUTRITION. (i) It’s a pleasure and a privilege to share it with you, the readers of my blogposts.
Table of contents
The autumn leaves harbor OPCs
The sight of leaves turning red in autumn is a spectacle to behold, particularly in Quebec, where thousands of maple trees turn crimson in the space of a few days. The leaves change colour so quickly that it is inconceivable that these trees, which are reaching the end of their vegetative period, can synthesise such a large quantity of pigment. We can therefore conclude that this phenomenon is not a question of creation, but rather the transformation of a colourless precursor. The mechanism behind this is still not fully understood, but in the laboratory we know how to extract these precursors from plant tissues and turn them red using a simple, rapid process. Proanthocyanidins (ii) are precisely this type of substance. Once isolated from the plant in colourless form, they can be converted into cyanidin, i.e., into the red pigments found in flowers and fruit. Proanthocyanidin simply means: cyanidin precursor. The intense colour produced captured the attention of chemists and has been the subject of a great deal of theoretical research. But the medicinal properties of proanthocyanidins are of even greater interest. The story of this discovery is well worth telling.
How were proanthocyanidins discovered?
It all began in 1945, when food shortages were still a major issue in France. Any new source of dietary protein was a welcome addition. One such source was groundnut cake, the industrial by-product of oil extraction usually only fed to livestock, which was considered for human consumption thanks to its amino acid content. We're all familiar with the thin reddish skin that encases peanuts. Should it be removed? Is the red pigment completely safe for human consumption? I was asked to investigate these questions. After isolating the pigment and confirming that it was harmless, I noticed that the skins contained a colourless substance that turned bright red when heated with an acid. The chemical structure of this precursor was similar to that of catechins, substances known at the time for their beneficial effects on the vascular system. In various animal tests, the colourless product proved to have the same properties, but to a much greater degree. I had just isolated the first proanthocyanidin and demonstrated its powerful protective effect on small blood vessels.
The plant kingdom therefore contained colourless constituents that were able to produce intensely red pigments, and which were also capable of curing certain patients’ susceptibility to haemorrhaging due to their fragile blood capillaries. In 1948, proanthocyanidin from peanuts started to be used therapeutically. Since then, various other plant sources (pine bark, grape seeds) have been used by the pharmaceutical industry to extract such substances. Millions of patients with vascular disorders have benefited from this medication, the safety of which has never been called into question.
Protectors against vascular disorders
Can we explain the mechanism behind the protective effect of proanthocyanidins? Initially, some biologists believed it was because the effects of adrenaline, a well-known vasoconstrictor, were prolonged. It was thought that the capillary would no longer ‘leak’ because its diameter would decrease and hold less circulating blood. However, this hypothesis was rejected because proanthocyanidins do not cause hypertension, which would be expected if adrenaline were involved. On the other hand, I was able to demonstrate that they have a strong affinity for collagen and elastin, major components of blood vessel walls, which they make both supple and resistant. When they bind to the blood vessel walls, proanthocyanidins improve the cohesion of the microscopic fibres that form the structure of these substances. In short, the ‘tube’ no longer leaks because its wall has become more solid. Finally, we were able to show that proanthocyanidins inhibit the excessive formation of histamine in the vascular walls. Histamine, which results from the biological breakdown of the amino acid histidine, increases the permeability of these walls. This is how gastric ulcers bleed, when stress, for example, causes histamine to be discharged from the stomach mucosa.
All of these factors combine to give proanthocyanidins a powerful vasculoprotective effect. For 40 years, this property alone was used for therapeutic purposes. Another chemical characteristic of the molecule, namely its antioxidant properties, was overlooked. In 1986, it was discovered that this characteristic gives proanthocyanidins a powerful scavenging effect on oxygenated free radicals (OFRs), which immediately attracted interested from the field of nutrition and dietetics.
Research into longevity
The experiments conducted in the USA by Harman in 1968 are the basis for our research into the scavenger effect. This biologist noted that adding BHT (butylhydroxytoluene) to the diet of mice increased their chances of survival. BHT is a product used in industry to protect various foodstuffs against the harmful effects of oxygen, one of which is the rancidification of fats. Harman used strains of mice carefully selected by cloning and which differed only in their survival threshold. With BHT, he was able to raise the threshold of mice normally destined for an early death by 45%, increasing their life expectancy to that of the more fortunate animals. It should be noted that longevity remained the same for all batches (around two years, a characteristic of the species). The only effect of BHT was the protection of at-risk animals from early death. At the time, Harman believed that these results could be transposed to humans by adding an antioxidant to the diet. Most biologists considered this view to be utopian. In any case, it was not possible to verify it for two reasons:
- among the known substances, no antioxidant was safe enough to be administered to humans on a daily and prolonged basis.
- the longevity of the human species exceeds a century, so the experiment would have to be continued for at least 50 years in order to assess the result.
What is the action of free radicals?
In the meantime, oxygenated free radicals (OFRs) had made their appearance in the field of biology. For a long time, radical reactions were considered to be incompatible with the fragility of living tissue due to their violence. They were only used in industrial chemistry. In 1969, Fridovich and McCord proved that radical oxygen, the superoxide anion, plays a role in cellular biochemistry. The impact of this discovery on medicine and biology in general was immense. The most recent development in this field concerns the use of OFR scavengers as medicines or food supplements.
To fully understand the nature of radical reactions, there is no need to get caught up in abstract physico-chemical discussions that are only of interest to specialists. Instead, let's look at an everyday example, such as a container of fat going rancid. It all starts on the surface, where the fat comes into contact with the air, and therefore with oxygen. But the oxygen in question is not present in its usual molecular form, O2. It has been discovered that cold and darkness slow down the process, whereas heat and light speed it up. This suggests that an initial input of energy (thermal, light) is required. This energy ‘boost’ is used to expel one of the electrons orbiting the oxygen nuclei. This loss of charge creates the intensely aggressive radical state that biochemists refer to as ‘the dark side of oxygen’. If you don't act quickly, the entire container of fat will go rancid. Oxidation takes place little by little; once it starts, there's no stopping it - it's a self-sustaining chain reaction. OFRs last for microseconds but, despite their transient nature, generate other radicals before disappearing. In fats, polyunsaturated fatty acids (PUFAs) are the target of choice for OFRs. The oils richest in PUFAs are therefore the most vulnerable. In industry, they are known as ‘siccatives’, which refers to the fact that they become less liquid under the action of oxygen.
How can the human body ‘go rancid’?
Can we extrapolate what we know about the container of fat to the human body? Curiously, dictionaries have long since answered this question with the word ‘rancidify’. Figuratively, it means to age by losing your qualities. For once, linguists and biochemists are in agreement. In fact, our bodies offer OFRs their favourite prey:
- polyunsaturated fatty acids, which can be found in all cell membranes, giving them the fluidity they need to perform their relational functions. Under the action of OFRs, membranes lose PUFAs, harden and no longer allow exchanges with the environment.
- connective tissue, where collagen and elastin are attacked, thus triggering accelerated ageing: loss of suppleness, resistance and capacity for hydration. This affects not only the skin, where the damage is clearly visible, but the entire circulatory system: capillaries, arteries, veins and lymphatic vessels.
- DNA, the carrier of the genetic code, is degraded by OFRs, leading to errors in protein synthesis.
- various ‘pre-carcinogenic’ molecules, which are not dangerous as they are, but which become so as a result of radical oxidation, thereby paving the way for the development of tumours.
- finally, many exogenous substances, brought in either by drugs or food, cannot be eliminated from our bodies in the usual ways and require the intervention of OFRs. They can be compared to bad fuel, such as damp wood, which only catches fire when excess dry wood is added. These include, on the one hand, synthetic organic molecules which are widely used in therapeutics, and on the other, alcohol, excessive quantities of which we know leads to the production of OFRs which act as 'scavengers’.
Safeguards against the action of free radicals
In a situation like this, it’s a wonder the human race has survived at all! Fortunately, nature has provided safeguards. It has equipped our cells with a set of enzymes (superoxide dismutase, catalase, glutathione peroxidase) responsible for reducing any excess OFRs. But because it is enzymatic by nature, this safeguard can fail. Firstly, because of the individual variations inherent in any genetic trait, and secondly because enzymes are proteins whose synthesis declines with age. It is therefore the elderly who are the least well protected, thus confirming the existence of individuals at risk from radical attacks.
A last resort is provided by our food, which contains various natural antioxidants (vitamin E, vitamin C) and trace elements (selenium) that boost the activity of protective enzymes. But dietary intake is random, which justifies our urgent search for truly reliable OFR captors. Several conditions must be met to fulfil this role:
- the captor must be harmless, even if used for prolonged periods.
- proven efficacy in vitro.
- availability in the body. This is an essential condition. It means that the captor, absorbed through the digestive tract, must be able to spread throughout the body to be present whenever and wherever a radical attack occurs.
In fact, of all the natural substances studied, only proanthocyanidins meet these criteria, as I demonstrated in 1986. We have long been aware of their safety and bioavailability. As OFR captors, their efficacy far exceeds that of the other substances I have tested comparatively in vitro. A year later, my results were confirmed by researchers at Nagasaki University. A proanthocyanidin studied in Japan proved to be 50 times more active than vitamin E, the reference substance for such tests. Finally, in 1988, I developed a process that enabled the scavenging effect of proanthocyanidins to be assessed in humans, thereby fulfilling the aspirations expressed by Harman 20 years previously.
Proanthocyanidins: new food supplements
The time has therefore come to use proanthocyanidins in food. Reserving their vascular effects for therapeutic use, it seems logical to me to regard these substances as dietary supplements that can make up for the lack of truly effective antioxidants in the diet. One might argue that proanthocyanidins, which are derived from the plant kingdom, are found in abundance in fruit and vegetables. Could it really be useful to make tablets from them? We know from experience that these constituents are found in very specific locations in plants: husks, bark, cuticles, seed coats, woody fibres - in other words, all the inedible parts we discard when preparing our meals.
Grapes are a good example: most proanthocyanidins are found in the seeds and are not present in the flesh. And even if we were to swallow the seeds, they do not allow their contents to be released into the digestive tract. Grape juice and white wine, obtained by pressing grapes, contain almost no proanthocyanidins. Red wine, on the other hand, contains high levels (500 mg per litre, for example), as the must ferments when it comes into contact with the seeds and the alcohol triggers their extraction.
Dietary indications for proanthocyanidins
What are the dietary indications for proanthocyanidins? These can be classified into three categories:
1. Diets rich in polyunsaturated fatty acids
We are all aware of the recent, and fully justified, popularity of food oils rich in polyunsaturated fatty acids (PUFAs), which are considered particularly useful in preventing atherosclerosis. Our bodies are unable to synthesise various so-called essential fatty acids, and the consumption of PUFAs bridges this gap. But we know that PUFAs are the preferred targets of OFRs and there is a good chance that these fatty acids will suffer the destructive effects of OFRs before reaching their destination in cells. If it were just for the perilous journey, the only disadvantage would be the waste. But lipid peroxidation, the complex process by which fatty acids are broken down, is accompanied by the production of a huge quantity of OFRs, some of which are much more toxic than the superoxide anion. Our natural defences struggle to keep this huge influx of radicals under control. Any dietary recommendation to consume PUFAs will trigger an influx of OFRs. A reliable captor must therefore be administered at the same time. By doing this, we are imitating nature, which only stores PUFAs alongside an antioxidant. Most often, it is a proanthocyanidin that performs this function, as is the case in peanuts, grape seeds, hazelnuts, etc.
2. The elderly
In the elderly, natural enzyme-based defences lose their effectiveness, making external support in the form of proanthocyanidins essential. Protecting connective tissue is imperative, not only to delay the ageing of the skin, but also to keep the vascular system as a whole in good working order.
3. Individuals at risk
Whether by necessity or habit, many people take risks with OFRs:
- by necessity, because they can't always escape external sources of OFRs, for example when spending extended periods in the sun or at high altitudes, as required by some professions. This also applies to patients treated with drugs such as synthetic hormones, steroids, antitumour agents and, in general, synthetic polycyclic molecules, since we know that these substances can only be broken down by a radical process.
- by habit, via tobacco and alcohol consumption. Smoking destroys vitamin C, thus depriving the body of a natural antioxidant. Proanthocyanidins bridge this gap and, thanks to their protective effect on ascorbic acid, make vitamin C more effective. Alcoholism and OFRs are directly related. Alcohol in quantities above the doses that are acceptable for the ADH (alcohol dehydrogenase) system in the liver must be eliminated by a radical pathway. Nordmann's work shows that organic disorders specific to alcoholics are the result of attacks by OFRs. It is therefore entirely appropriate to prescribe proanthocyanidins to heavy drinkers. The validity of this practice can be deduced from the most rigorous epidemiological data available worldwide. The mortality rate from alcoholism is always lower (by a factor of 2 to 4) in populations that drink their alcohol in the form of red wine, compared with those that drink it in the form of white wine or any other alcoholic beverage. The proanthocyanidins in red wine undeniably play a protective role. Needless to say, this only applies to moderate consumption. Due to the chain extension of radical processes, alcohol drunk in excess outweighs the capturing effect of proanthocyanidins, which is expressed in a linear fashion.
The plant world has plenty more surprises in store for us
To conclude this brief review of proanthocyanidins, two remarks must be made. First of all, we have to acknowledge that therapeutics has been dominated by synthetic chemistry for half a century. Although most of the successes in the art of healing have been inspired by models of botanical origin, the synthetic molecule instils more confidence than the undefined concoctions of herbal tea aficionados. At least that's the official line. After all, the general public is constantly showing its support for ‘alternative’ medicines, a term which implies that these wonderful molecules are not always very gentle and that their side-effects can become formidable. Proanthocyanidins show that the plant world still has some pleasant surprises in store for us, combining effectiveness with safety. In this particular case, we can see that a natural remedy is capable of repairing the damage caused by a number of products of organic synthesis. My second comment risks being labelled chauvinistic. The effects of proanthocyanidins on the human body were discovered in France, and our country is a leader in this field. I hope that the French will continue to be at the forefront of dietetic applications and that, for once, our knowledge does not come from overseas.
Prof. Jack Masquelier / Martillac, France / 1990
+ + + + + + + + +
[i] In the original French text, Masquelier uses the term “procyanidols”. In the contaxt of the article, “procyanidols” (translated as: procyanidins) must be understood as meaning OPCs.
[ii] PROANTHOCYANIDINS: SUBSTANCES THAT FIGHT FREE RADICALS; Prof. Jack Masquelier, Evolutionary Nutrition; Quarterly Jpoiurnal, No. 11, 3rd Quarter 1990; Éditions RETZ, Paris.